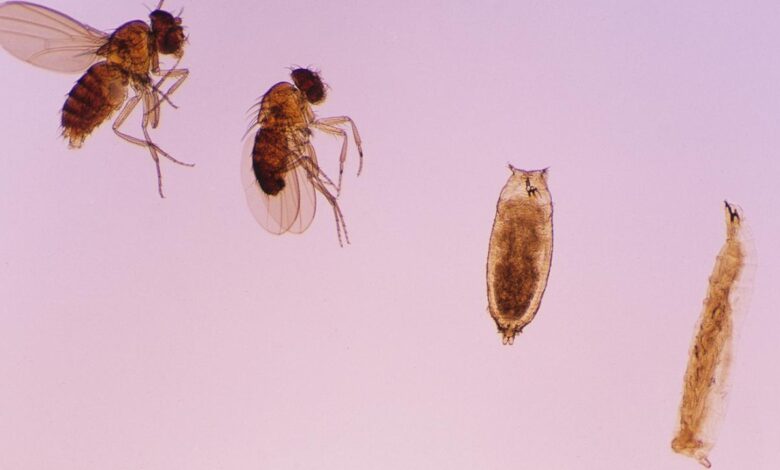
A Fruit Fly Brain Map Understanding Our Own
A map of a fruit flys brain could help us understand our own – A map of a fruit fly’s brain could help us understand our own – it sounds crazy, right? But the truth is, these tiny creatures share surprisingly similar brain structures and functions with humans. Think about it: we’re both capable of learning, remembering, and even exhibiting complex behaviors. By meticulously mapping the fruit fly brain, scientists are unlocking secrets about how our own brains work, paving the way for breakthroughs in treating neurological disorders and even enhancing our understanding of consciousness itself.
This isn’t just about tiny insects; it’s about the fundamental building blocks of intelligence.
The research involves cutting-edge imaging techniques, allowing scientists to visualize the intricate neural pathways within the fruit fly brain. This detailed map reveals how neurons connect and communicate, providing insights into the complex processes that underpin our thoughts, actions, and memories. The challenges are immense, involving incredibly high-resolution imaging and the integration of data from multiple sources. But the potential rewards – a deeper understanding of our own brains – are simply too significant to ignore.
Similarities Between Fruit Fly and Human Brains
The seemingly vast difference in size and complexity between a fruit fly’s brain and a human brain belies a surprising degree of similarity at a fundamental level. Decades of research have revealed striking parallels in neural structures, functionalities, and even the genetic blueprints underlying their development. Understanding these similarities provides invaluable insights into the basic principles governing brain function and offers powerful model systems for studying human neurological diseases.
Fundamental Neural Structures
Both fruit fly and human brains are built upon a common framework of neurons, synapses, and glial cells. While the sheer number of these components differs dramatically, the basic architecture—the way neurons connect and communicate—shares remarkable conservation. For instance, both species possess distinct brain regions responsible for processing sensory information (vision, smell, touch), motor control, and higher-order cognitive functions (though the sophistication of these functions varies considerably).
This underlying structural similarity suggests that the fundamental principles of neural computation are ancient and highly conserved throughout evolution.
It’s amazing how studying the surprisingly complex brain of a fruit fly could unlock secrets about our own. The intricate neural pathways offer fascinating insights into fundamental brain processes. Meanwhile, in completely unrelated news, the ongoing legal drama surrounding former President Trump took another turn when, as reported in this article about the judge blocking the special master , security concerns overshadowed other aspects of the case.
Understanding the complexities of even a tiny insect brain, however, highlights the immense challenge of deciphering the human one.
Basic Functionalities of Shared Structures
Despite the differences in scale, many fundamental neural processes are remarkably similar. Neurotransmission, for example, relies on the same basic mechanisms in both fruit flies and humans. Neurotransmitters like glutamate and GABA play crucial roles in excitatory and inhibitory signaling in both species. Similarly, the basic mechanisms of synaptic plasticity—the ability of synapses to strengthen or weaken over time—are conserved, although the complexity and regulation of these processes are more intricate in humans.
Learning and memory, fundamental processes relying on synaptic plasticity, have been extensively studied in fruit flies, providing valuable insights into the underlying mechanisms in humans.
Evolutionary Conservation of Neural Pathways
The evolutionary conservation of neural pathways is strikingly evident in the shared genetic basis of brain development. Many genes involved in neuronal differentiation, axon guidance, and synapse formation are highly conserved between fruit flies and humans. This conservation suggests that the fundamental mechanisms of brain development evolved early in animal evolution and have been retained throughout subsequent diversification.
Studying these conserved pathways in the simpler fruit fly brain provides a tractable model for understanding the complex processes of human brain development and the potential causes of developmental disorders.
It’s mind-blowing how mapping a fruit fly’s brain, with its surprisingly complex neural pathways, could unlock secrets about our own. The sheer intricacy of even a tiny insect’s mind makes you wonder about the ethical implications of power, like the news that the Biden administration might grant immunity to the Saudi Crown Prince in the Khashoggi murder lawsuit, as reported here: biden admin suggests saudi crown prince be granted immunity in khashoggi murder lawsuit.
Understanding these vastly different scales of complexity – from a fruit fly’s brain to international geopolitics – really makes you think about the nature of decision-making itself.
Genes and Proteins Involved in Brain Development
Numerous genes and proteins are involved in brain development in both fruit flies and humans. For example, the
- Pax6* gene, crucial for eye development, is highly conserved and plays a similar role in both species. Similarly, genes involved in neurogenesis, such as
- Notch* and
- Delta*, are conserved and regulate the production of new neurons in both fruit fly and human brains. Furthermore, many signaling pathways, such as the Wnt and Hedgehog pathways, are crucial for patterning and development of the brain in both species. The conservation of these genes and proteins highlights the deep evolutionary relationship between fruit fly and human brains.
Comparison of Brain Region Size and Complexity
Brain Region | Fruit Fly | Human |
---|---|---|
Number of Neurons | ~100,000 | ~86 billion |
Optic Lobes (Visual Processing) | Relatively large proportion of brain | Relatively small proportion of cortex |
Mushroom Bodies (Learning & Memory) | Complex structure crucial for learning | Analogous functions distributed across hippocampus and other areas |
Cerebral Cortex (Higher-order processing) | Absent | Highly developed, responsible for complex cognitive functions |
Mapping the Fruit Fly Brain
Mapping the fruit fly brain, despite its seemingly small size, presents a significant challenge and a remarkable opportunity for neuroscience. Its relative simplicity compared to the human brain, coupled with the wealth of genetic tools available, makes it an ideal model organism for understanding fundamental neural processes. A complete and accurate map of the fruit fly brain could unlock secrets about brain function and organization applicable to more complex brains, including our own.
Imaging Techniques for Fruit Fly Brain Mapping, A map of a fruit flys brain could help us understand our own
Several advanced imaging techniques are employed to create detailed maps of the fruit fly brain. These techniques provide complementary information, allowing for a more holistic understanding of brain structure and connectivity. Confocal microscopy, for instance, allows for the visualization of fluorescently labeled neurons, revealing their morphology and spatial relationships. Electron microscopy, on the other hand, offers significantly higher resolution, allowing researchers to visualize individual synapses and neuronal structures at a nanometer scale.
Techniques like serial block-face scanning electron microscopy (SBFSEM) automate the process of imaging large volumes of tissue, greatly accelerating data acquisition. Finally, techniques like diffusion tensor imaging (DTI) can map the white matter tracts and connectivity pathways within the brain.
Challenges in High-Resolution Brain Mapping
Creating a comprehensive, high-resolution map of the fruit fly brain faces numerous hurdles. The sheer volume of data generated by techniques like electron microscopy is immense, requiring powerful computational resources for processing and analysis. Another challenge lies in the inherent complexity of the brain itself. The intricate three-dimensional arrangement of neurons, their axons, dendrites, and synapses presents a significant challenge for accurate reconstruction.
Furthermore, aligning and stitching together images from different sections of the brain to create a complete three-dimensional model requires sophisticated image registration algorithms. Finally, variations between individual fruit fly brains necessitate the analysis of multiple brains to establish a representative map.
Data Integration from Multiple Imaging Modalities
Integrating data from different imaging modalities is crucial for a complete understanding of the fruit fly brain. For example, confocal microscopy data can provide an overview of neuronal populations and their general organization, while electron microscopy data can reveal the detailed circuitry at the synaptic level. Computational methods are essential for aligning and merging this heterogeneous data. Sophisticated algorithms are used to register images from different modalities and create a unified, multi-scale representation of the brain.
This integrated approach allows researchers to link the macroscopic organization of the brain with its microscopic circuitry.
Limitations of Current Mapping Technologies and Future Improvements
Current mapping technologies, while advanced, still have limitations. The time and resources required for high-resolution electron microscopy are substantial, limiting the scale of studies. Furthermore, current methods struggle to capture dynamic aspects of brain activity, such as changes in synaptic strength or neuronal firing patterns. Future improvements may involve the development of faster and more efficient imaging techniques, coupled with advancements in computational tools for data processing and analysis.
The integration of other “omics” data, such as genomics and transcriptomics, will provide a more comprehensive understanding of brain structure and function. The development of automated methods for neuron tracing and synapse identification is also crucial.
Hypothetical Experiment to Address a Specific Limitation
One major limitation is the difficulty in capturing the dynamic aspects of neuronal activity. A hypothetical experiment to address this could involve combining high-resolution electron microscopy with techniques for monitoring neuronal activity in vivo. For example, genetically encoded calcium indicators could be expressed in specific neuronal populations, allowing for the simultaneous visualization of neuronal structure and activity. By imaging the same brain region using both electron microscopy and calcium imaging at different time points, researchers could correlate structural features with dynamic changes in neuronal activity.
This would provide insights into how changes in synaptic connections or neuronal firing patterns influence behavior and cognitive processes. This experiment could be performed by initially creating a transgenic fly line expressing a calcium indicator, then imaging its brain at rest and during a specific behavioral task, using both electron microscopy and calcium imaging techniques to compare the two datasets.
Fruit Fly Brain Map Applications in Neuroscience Research: A Map Of A Fruit Flys Brain Could Help Us Understand Our Own
The creation of a detailed map of the fruit fly brain,Drosophila melanogaster*, represents a monumental achievement in neuroscience. This intricate map, providing unprecedented resolution of neural connections, has opened up exciting new avenues for research and promises to significantly advance our understanding of the brain, both in flies and, remarkably, in humans. Its applications extend across various domains of neuroscience, offering powerful tools for investigating fundamental biological processes and developing new therapeutic strategies.
The fruit fly’s relatively simple nervous system, while sharing remarkable similarities with more complex brains, makes it an ideal model organism for studying fundamental neural processes. Its genetic tractability and the ability to manipulate its neural circuits with precision allow researchers to unravel complex biological mechanisms in a way that is simply not feasible in more complex systems, like the human brain.
Advances in Understanding Neural Circuits
The detailed map of theDrosophila* brain has already provided crucial insights into the organization and function of neural circuits. For example, researchers have used the map to trace specific neuronal pathways involved in behaviors such as learning, memory, and sensory processing. By manipulating specific neurons identified within the map, scientists can directly observe the effects on behavior, providing a powerful causal link between neural activity and function.
This has allowed for a much more nuanced understanding of how different brain regions interact to produce complex behaviors, knowledge that is directly transferable to the study of analogous circuits in the human brain.
Implications for Understanding Neurological Disorders in Humans
The striking conservation of many fundamental neural mechanisms between flies and humans makes the fruit fly brain map invaluable for studying neurological disorders. Many genes implicated in human neurological diseases, such as Parkinson’s disease and Alzheimer’s disease, have functional homologs inDrosophila*. By studying the effects of mutations in these genes on the fly brain, researchers can gain insights into the underlying mechanisms of these diseases.
Mapping a fruit fly’s brain, believe it or not, might unlock secrets about our own complex neural networks. It’s all about finding commonalities in neural pathways, and sometimes the unexpected connections surprise you, much like the market reaction to the recent Labour budget; check out this article on how labours budget has given the bond market indigestion for a parallel example of complex systems reacting in unpredictable ways.
Understanding these intricate systems, whether it’s a tiny fly brain or the global economy, requires similar analytical approaches.
The map provides a framework for identifying specific neural circuits affected by these mutations, paving the way for the development of targeted therapies. For instance, researchers can pinpoint specific neuronal populations vulnerable to degeneration in models of Parkinson’s disease, leading to a more focused search for effective treatments.
Studying the Effects of Drugs and Other Interventions
The fruit fly brain map facilitates the study of how drugs and other interventions affect neural circuits. Researchers can use the map to identify specific neurons targeted by a drug and observe its effects on neuronal activity and behavior. This approach allows for a more precise understanding of a drug’s mechanism of action and can help to identify potential side effects.
Furthermore, the map can be used to screen for new drugs that target specific neural circuits implicated in neurological disorders, accelerating the drug discovery process. This high-throughput screening, facilitated by the map’s detailed anatomical information, allows for the testing of numerous compounds in a relatively short timeframe.
Contribution to the Development of New Therapies for Neurological Diseases
The fruit fly brain map is a crucial tool for developing new therapies for neurological diseases. By identifying specific neural circuits involved in a disease, researchers can develop targeted therapies that aim to restore normal function. This could involve gene therapy, where specific genes are manipulated to correct faulty neural circuits, or the development of new drugs that target specific neural pathways.
The detailed anatomical information provided by the map allows for the precise delivery of therapeutic agents to the affected areas, increasing the efficacy of the treatment and reducing potential side effects. For example, research using the fly model has already yielded promising results in the development of novel therapies for neurodegenerative diseases.
Potential Applications of the Fruit Fly Brain Map in Diverse Areas of Neuroscience
The applications of the fruit fly brain map extend far beyond the examples discussed above. The map is a powerful resource for studying a wide range of neuroscience questions, including:
The map’s detailed anatomical information provides a crucial foundation for numerous research endeavors. Its impact on our understanding of the brain, and the development of new therapies for neurological diseases, is likely to be profound and far-reaching.
Ethical Considerations and Societal Impact
The remarkable progress in mapping the fruit fly brain opens exciting avenues for understanding the human brain, but it also raises significant ethical considerations and societal implications. Translating findings from a simple invertebrate model to the complex human brain requires careful consideration of potential risks and benefits, and necessitates a robust ethical framework to guide research and application. The potential for both immense progress and unforeseen consequences necessitates a thoughtful and nuanced discussion.The use of fruit flies as a model organism in neuroscience research presents a unique ethical landscape.
While fruit flies lack the complex cognitive abilities and sentience of humans, ethical considerations still apply. Minimizing suffering and ensuring humane treatment during experiments are paramount. The ethical debate centers around the balance between the potential benefits of scientific advancement and the welfare of the organisms used in research. This balance is further complicated by the potential for misinterpretation or over-extrapolation of findings from fruit fly research to humans, leading to potentially harmful consequences.
Ethical Implications of Using Fruit Fly Brain Research to Understand Human Brains
The primary ethical concern revolves around the potential for anthropomorphism – the attribution of human characteristics to non-human entities. While fruit fly brains share some structural and functional similarities with human brains, they are fundamentally different. Oversimplifying these differences and drawing overly direct parallels could lead to flawed conclusions about human cognition, behavior, and disease. This could, in turn, influence policy decisions or therapeutic interventions based on incomplete or inaccurate information.
Another concern is the potential for misallocation of resources. While fruit fly research is cost-effective and efficient, over-reliance on this model might divert funding from other potentially fruitful avenues of neuroscience research.
Societal Impact of Advancements in Neuroscience Stemming from Fruit Fly Brain Mapping
Advancements in neuroscience, fueled by fruit fly brain mapping, hold immense potential for improving human health and well-being. For instance, a deeper understanding of neurological diseases like Parkinson’s or Alzheimer’s could lead to more effective diagnostic tools and treatments. Furthermore, this research could contribute to the development of novel therapies for cognitive impairments and mental health disorders. However, societal impact extends beyond medical applications.
Insights into brain function could revolutionize fields like education, artificial intelligence, and even criminal justice. For example, a better understanding of learning and memory could lead to more effective educational strategies.
Potential Risks and Benefits Associated with Translating Research Findings from Fruit Flies to Humans
Translating research findings from fruit flies to humans carries both substantial benefits and significant risks. Benefits include the potential for faster and cheaper drug discovery, leading to quicker development of treatments for neurological disorders. Improved understanding of fundamental brain processes could revolutionize our understanding of consciousness and cognition. Risks include inaccurate extrapolations of findings, leading to ineffective or even harmful treatments.
The potential for misinterpretation and over-simplification necessitates cautious and rigorous validation of findings in more complex animal models and ultimately, human clinical trials. The ethical implications of translating findings from a simple invertebrate to a complex human system must be meticulously considered at each stage of the research process.
Comparison of Ethical Considerations of Using Animal Models in Neuroscience Research to Other Approaches
The use of animal models, including fruit flies, in neuroscience research raises ethical questions about the welfare of the animals. These concerns must be weighed against the potential benefits to human health. Alternative approaches, such as in vitro studies using human cells or computational modeling, offer ethical advantages by avoiding the use of animals. However, these methods have limitations in their ability to capture the complexity of the living brain.
The ethical choice often involves balancing the potential benefits of research with the ethical obligations to minimize harm to animals, while simultaneously acknowledging the limitations of alternative approaches.
Societal Benefits and Challenges Arising from Fruit Fly Brain Mapping Research
The potential societal benefits and challenges associated with this research are multifaceted and far-reaching.
- Benefits: Improved understanding and treatment of neurological and psychiatric disorders; advancements in artificial intelligence and robotics; enhanced educational strategies; development of novel brain-computer interfaces.
- Challenges: Potential for misuse of neuroscience findings; ethical concerns surrounding genetic manipulation and enhancement; widening health disparities due to unequal access to advanced treatments; societal anxieties regarding the manipulation of human consciousness.
Visual Representation of Fruit Fly Brain Mapping Data
Visualizing the intricate neural network of a fruit fly brain presents a significant challenge, given its complexity and the sheer volume of data generated through mapping techniques. Effective visualization is crucial not only for researchers to understand the data but also for communicating these findings to a wider scientific community and the public. Various methods exist, each with its strengths and limitations, allowing researchers to explore and present this complex data in accessible and informative ways.The process of visualizing fruit fly brain mapping data involves transforming raw data, such as connectivity matrices and neuron locations, into comprehensible visual representations.
This can range from simple 2D diagrams to sophisticated interactive 3D models. The choice of visualization method depends heavily on the specific research question and the type of data being analyzed.
Three-Dimensional Modeling of the Fruit Fly Brain
Creating a 3D model of a fruit fly brain leverages the spatial coordinates of neurons and their connections obtained through techniques like confocal microscopy and automated tracing algorithms. Software packages like Blender, 3D Slicer, or custom-built solutions are used to construct these models. The models can be color-coded to represent different brain regions, neuron types, or connection strengths.
For instance, a model might show the mushroom body, a crucial region for learning and memory, in a vibrant green, while the optic lobes, responsible for visual processing, are depicted in blue. The thickness of connections between neurons can also be modulated to represent the strength of synaptic transmission, providing a visual representation of information flow within the brain.
These models allow for interactive exploration, enabling researchers to rotate, zoom, and dissect the virtual brain to investigate specific neural circuits in detail. Such visualizations are far more intuitive than looking at tables of numerical coordinates.
Importance of Clear and Effective Data Visualization in Communicating Research Findings
Clear and effective data visualization is paramount in neuroscience research for several reasons. Firstly, it facilitates understanding. Complex datasets, such as those from brain mapping studies, can be overwhelming in their raw form. Visualizations transform abstract data into easily digestible formats, enabling researchers to identify patterns, anomalies, and relationships that might be missed through purely numerical analysis. Secondly, visualization enhances communication.
Sophisticated 3D models and interactive visualizations can be incorporated into presentations, publications, and online resources, making complex research accessible to a broader audience, including non-specialists. Finally, effective visualizations promote collaboration. Shared visual representations provide a common ground for researchers to discuss findings, identify areas of agreement and disagreement, and foster collaborative research efforts.
Examples of Effective Visual Representations in Neuroscience
Several established visualization techniques have proven effective in neuroscience. Heatmaps are frequently used to display the density or intensity of neural activity across different brain regions. Network graphs, showing neurons as nodes and connections as edges, are useful for visualizing the overall architecture of neural circuits. Interactive 3D models, as discussed previously, allow for a more immersive exploration of the brain’s structure and function.
Furthermore, virtual reality (VR) environments are increasingly being used to explore large-scale brain datasets, providing an unparalleled level of immersion and interactivity. The selection of the most appropriate method depends on the nature of the data and the research question being addressed.
Hypothetical Image of a Specific Fruit Fly Brain Region
Imagine a high-resolution image of the Kenyon cells within the fruit fly’s mushroom body. The image is a 3D rendering, colored according to the expression level of a specific gene associated with learning and memory. Kenyon cells exhibiting high gene expression are rendered in a bright, saturated yellow, while those with lower expression are depicted in a progressively paler yellow, down to a light beige.
The image also shows the intricate dendritic arborizations of these cells, highlighting their extensive interconnections. Fine, almost translucent blue lines represent the axons of other neurons projecting into the mushroom body, forming synapses with the Kenyon cells. The background is a dark grey, emphasizing the Kenyon cells and their connections. This image allows researchers to visually assess the distribution and intensity of gene expression within this critical brain region, potentially revealing insights into the molecular mechanisms underlying learning and memory.
The journey to map the fruit fly brain is a testament to human ingenuity and our relentless pursuit of knowledge. While still in its early stages, this research promises to revolutionize our understanding of the human brain. From developing new therapies for neurological diseases to gaining a deeper appreciation of the complexities of consciousness, the implications are far-reaching.
The fruit fly, a seemingly insignificant creature, may hold the key to unlocking some of the biggest mysteries of the human mind. It’s a truly humbling and awe-inspiring prospect.