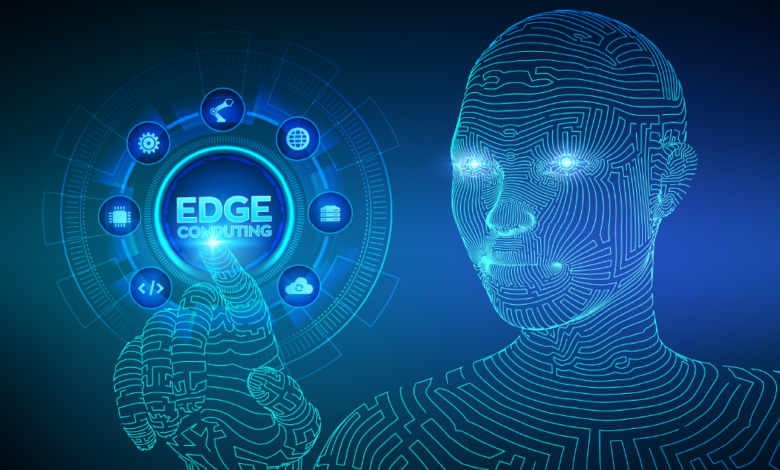
Researchers Are Looking Beyond Digital Computing
Researchers are looking beyond digital computing, and it’s a fascinating journey! We’re on the cusp of a revolution, moving beyond the silicon chips that have powered our technological advancements for decades. This exploration delves into the exciting alternatives emerging – from the mind-bending potential of quantum computing to the bio-inspired elegance of DNA computing and the physics-based approaches using light and sound.
Get ready to explore the future of computation!
This isn’t just about faster processors; it’s about fundamentally different ways of solving problems. Imagine computers inspired by the human brain, or algorithms encoded in DNA strands. These emerging technologies promise breakthroughs in areas where digital computing struggles, offering solutions to problems that are currently intractable. We’ll explore the unique strengths and weaknesses of each approach, examining their potential applications and the challenges involved in their development.
Alternative Computing Paradigms
The limitations of classical digital computing, particularly in tackling complex problems like protein folding or materials science simulations, are driving the exploration of alternative computing paradigms. These non-digital approaches leverage different physical phenomena to perform computations, offering potential advantages in specific domains where traditional methods fall short. This exploration delves into three prominent examples: quantum computing, neuromorphic computing, and DNA computing.
Quantum Computing
Quantum computing harnesses the principles of quantum mechanics, specifically superposition and entanglement, to perform computations. Unlike classical bits representing 0 or 1, quantum bits (qubits) can exist in a superposition of both states simultaneously. This allows quantum computers to explore multiple possibilities concurrently, potentially solving certain problems exponentially faster than classical computers. Entanglement further enhances this capability by linking the fates of multiple qubits, creating powerful correlations.
Current quantum computers are still in their early stages, facing challenges in maintaining qubit coherence and scaling up the number of qubits. However, promising applications include drug discovery (simulating molecular interactions), materials science (designing new materials with specific properties), and cryptography (breaking current encryption methods). Companies like IBM and Google are actively developing quantum computing hardware and software, while research institutions worldwide are pushing the boundaries of this technology.
Neuromorphic Computing
Inspired by the structure and function of the human brain, neuromorphic computing utilizes artificial neural networks implemented in hardware. These systems mimic the brain’s parallel processing capabilities, enabling efficient handling of complex, unstructured data. Unlike traditional computers relying on sequential instructions, neuromorphic chips process information in a distributed and parallel manner, consuming significantly less energy. The hardware itself is designed to mimic the behavior of neurons and synapses, leading to improved energy efficiency and real-time processing.
Potential applications include image recognition, natural language processing, and robotics, where real-time processing and adaptation to changing environments are crucial. Companies like Intel and IBM are leading the development of neuromorphic chips, with applications already emerging in areas like autonomous driving and smart sensors.
DNA Computing
DNA computing leverages the inherent computational power of DNA molecules. By encoding information into DNA sequences and using biochemical reactions to manipulate and process this information, it’s possible to solve complex combinatorial problems. This approach offers massive parallelism, as billions of DNA strands can be processed simultaneously. However, DNA computing is limited by the speed of biochemical reactions and the difficulty of error correction.
Despite these challenges, DNA computing holds promise for solving specific computationally hard problems, such as finding optimal routes or designing complex molecules. Research in this area is still largely experimental, but potential applications include drug discovery, materials science, and solving complex optimization problems that are intractable for classical computers.
Comparative Analysis of Computing Paradigms
The following table compares the scalability, energy efficiency, and computational power of different computing methods. Note that these are broad generalizations, and the specific performance of each technology depends heavily on the specific implementation and problem being addressed.
Feature | Digital Computing | Quantum Computing | Neuromorphic Computing | DNA Computing |
---|---|---|---|---|
Scalability | High (currently) | Low (limited by qubit coherence) | Moderate (growing rapidly) | Low (limited by biochemical processes) |
Energy Efficiency | Moderate (improving) | Low (high energy consumption per qubit) | High (parallel processing) | High (inherent parallelism) |
Computational Power | High for specific tasks | Potentially exponential advantage for specific problems | High for parallel tasks and pattern recognition | High for specific combinatorial problems |
Biological and Chemical Computing: Researchers Are Looking Beyond Digital Computing
The digital revolution has undeniably transformed our world, but the limitations of silicon-based computing are becoming increasingly apparent as we grapple with problems of ever-increasing complexity. This has spurred exploration into alternative computing paradigms, and among the most promising are those leveraging the power of biological and chemical systems. These approaches offer the potential for massively parallel processing, energy efficiency, and the ability to tackle problems currently intractable for even the most powerful supercomputers.Biological systems, with their inherent complexity and sophistication, provide a rich source of inspiration for novel computational approaches.
DNA computing, in particular, stands out as a compelling example of this potential.
DNA Computing Principles and Problem-Solving Capabilities
DNA computing harnesses the natural properties of DNA molecules – their ability to store information, replicate, and undergo specific reactions – to perform computations. Instead of using binary digits (0s and 1s), DNA computing utilizes the four nucleotide bases (adenine, guanine, cytosine, and thymine) to encode information. Complex computations are performed through carefully designed biochemical reactions, such as hybridization (the binding of complementary DNA strands) and enzymatic cleavage (cutting DNA strands at specific locations).
This allows for massive parallelism, as billions of DNA molecules can undergo reactions simultaneously, potentially solving complex optimization problems, such as the Traveling Salesperson Problem, far more efficiently than traditional digital computers. For instance, Leonard Adleman’s pioneering experiment in 1994 demonstrated the feasibility of solving a small instance of the Hamiltonian path problem using DNA. This groundbreaking work paved the way for further research into the potential of DNA computing to tackle larger and more intricate problems.
It’s fascinating how researchers are looking beyond digital computing, exploring quantum and biological alternatives. This push for innovation comes at a time when we’re grappling with major societal issues, like the recent attack on Paul Pelosi, where officials have confirmed the alleged paul pelosi attacker is an illegal immigrant officials confirm. Such events highlight the need for advancements, not just in technology, but in understanding complex societal problems that impact our lives.
The search for new computing paradigms might just offer solutions we haven’t even imagined yet.
Challenges and Limitations of Biological Computing
Despite its potential, DNA computing faces significant challenges. Error rates in biochemical reactions can be relatively high, leading to inaccurate results. The design and synthesis of DNA strands for specific computations can be complex and time-consuming. Furthermore, scaling up DNA computations to handle truly massive problems remains a significant hurdle. The inherent limitations of diffusion and reaction kinetics in biological systems also pose challenges for the speed and efficiency of computations.
Controlling and manipulating large numbers of DNA molecules accurately and efficiently is another major technological challenge. Finally, the cost and expertise required to perform DNA computations are currently quite high, limiting accessibility.
Examples of Bio-inspired Computing Research and Impact
Research in bio-inspired computing extends beyond DNA computing. Scientists are exploring the use of other biological molecules, such as RNA and proteins, for computation. For example, research into ribosome-based computing aims to leverage the protein synthesis machinery of cells to perform computations. Additionally, bio-inspired computing extends to designing artificial neural networks that mimic the structure and function of the human brain, leading to advancements in artificial intelligence.
These bio-inspired approaches have potential impacts across diverse fields, including drug discovery, materials science, and environmental monitoring. For example, bio-inspired algorithms are used in optimizing drug delivery systems, designing novel materials with specific properties, and developing more efficient sensors for environmental pollutants.
It’s fascinating how researchers are looking beyond digital computing, exploring quantum and biological alternatives. This reminds me of the recent controversy surrounding Alexandria Ocasio-Cortez, where a review of ocasio cortezs campaign finance records website contradict claims that merchandise profits go to charity , highlighting the complexities of transparency even in seemingly straightforward areas. The need for clear accounting, much like the need for reliable computing, is paramount in our increasingly complex world.
Ultimately, both fields require rigorous verification and innovative solutions.
Types of Bio-molecular Computing, Researchers are looking beyond digital computing
The following table summarizes different types of bio-molecular computing, highlighting their advantages and disadvantages:
Type | Advantages | Disadvantages |
---|---|---|
DNA Computing | Massive parallelism, inherent error correction mechanisms, potential for high energy efficiency | High error rates, complex design and synthesis, scaling challenges, cost and expertise requirements |
RNA Computing | Similar to DNA computing, but RNA’s catalytic activity offers additional computational possibilities | Similar to DNA computing, but also susceptibility to RNA degradation |
Protein Computing | Potential for high specificity and catalytic activity, ability to perform complex chemical transformations | Complex design and synthesis, challenges in controlling protein folding and interactions |
Ribosome-based Computing | Leverages the natural protein synthesis machinery for computation | Challenges in programming and controlling the ribosome’s activity |
Physical Phenomena for Computation
The digital revolution has been built on the manipulation of electrons, but the quest for faster, more energy-efficient computation is pushing researchers to explore alternative paradigms. Harnessing the inherent computational capabilities of physical phenomena like light and sound offers exciting possibilities for overcoming the limitations of traditional silicon-based computing. These approaches leverage the unique properties of these phenomena to perform computations in novel ways, potentially leading to breakthroughs in various fields.
Researchers are actively investigating how the properties of light, sound, and other physical phenomena can be exploited for computational tasks. Optical computing, for instance, uses photons instead of electrons to represent and process information. This approach can offer significant advantages in terms of speed and bandwidth, as photons can travel much faster and carry more information than electrons.
Similarly, acoustic computing leverages sound waves to perform computations, offering unique advantages in specific applications.
Optical Computing
Optical computing utilizes light for information processing. Photons, unlike electrons, don’t interact strongly with each other, leading to reduced crosstalk and the potential for massively parallel processing. Optical logic gates, analogous to transistors in electronic circuits, can be implemented using various optical components such as lasers, waveguides, and interferometers. However, challenges remain in creating efficient and scalable optical interconnects and memory devices.
Current research focuses on developing novel materials and architectures to overcome these limitations. One example of an optical computing application is high-speed data transmission in telecommunications networks, where the immense bandwidth of optical fibers is exploited.
Acoustic Computing
Acoustic computing, in contrast to optical computing, uses sound waves to process information. This approach offers unique advantages in specific environments, such as underwater or in harsh conditions where electronic components might fail. Acoustic waves can propagate through various media, allowing for computations to be performed in diverse environments. The development of acoustic logic gates and memory elements is an active area of research.
It’s fascinating how researchers are looking beyond digital computing for new solutions, exploring everything from quantum mechanics to bio-computing. This reminds me of how drastically our culture has shifted, as evidenced by the impact of weight loss drugs have changed culture ; it shows how rapidly societal norms can evolve around readily available technological and medical advancements.
Ultimately, this drive for innovation, whether in computing or medicine, reflects our constant search for better ways of life.
Acoustic computing is particularly promising for applications requiring low power consumption and robustness, such as underwater sensing and environmental monitoring. Imagine a network of underwater sensors communicating and processing data using sound waves – this is a potential application of acoustic computing.
Applications of Physical Computing Methods
Physical computing methods, encompassing both optical and acoustic approaches, hold immense potential across numerous applications. In sensing, optical sensors can detect minute changes in light intensity or polarization, enabling highly sensitive measurements. Acoustic sensors can be used for underwater detection, non-destructive testing, and medical imaging. In imaging, optical techniques like optical coherence tomography (OCT) provide high-resolution images of biological tissues.
In signal processing, optical and acoustic methods can be used for high-speed data processing and filtering, crucial for applications like radar and sonar systems.
Comparison of Physical Computing Approaches
The following table compares the speed, accuracy, and energy consumption of various physical computing approaches. Note that these are general comparisons and specific performance can vary greatly depending on the implementation and technology used.
Computing Method | Speed | Accuracy | Energy Consumption |
---|---|---|---|
Optical Computing | Very High | High | Moderate to High (depending on implementation) |
Acoustic Computing | Moderate | Moderate to High | Low |
Electronic Computing (for comparison) | High | High | Moderate |
The Future of Computing Architectures
The relentless pursuit of faster, more efficient, and more powerful computing has led researchers to explore alternatives beyond the limitations of traditional digital architectures. While digital computing remains dominant, the horizon is brimming with possibilities offered by the convergence of diverse computing paradigms. This convergence promises to unlock computational capabilities previously deemed unattainable, ushering in a new era of problem-solving across various scientific and technological domains.The integration of different computing paradigms, such as digital, quantum, and biological computing, is no longer a futuristic fantasy but an active area of research and development.
This hybrid approach leverages the strengths of each paradigm, compensating for their individual weaknesses and creating systems with enhanced performance and capabilities. For instance, the inherent parallelism of biological systems can be combined with the precision of digital circuits, while the quantum realm’s potential for solving intractable problems can be integrated to enhance overall computational power.
Hybrid Computing System Examples
Research into hybrid systems is yielding exciting results. One prominent area focuses on integrating digital controllers with quantum annealing processors. Digital circuits manage the input and output, preparing data for the quantum processor and interpreting its results. This allows researchers to harness the power of quantum mechanics for specific tasks while relying on the established reliability and scalability of digital components.
Another example involves using biological molecules like DNA to perform computations, with digital systems managing the process and interpreting the outcomes. This approach explores the potential of massively parallel biological computations for tasks such as drug discovery and materials science. Furthermore, researchers are investigating the integration of neuromorphic chips (inspired by the human brain’s architecture) with classical digital processors for applications requiring both high-speed computation and sophisticated pattern recognition.
These hybrid systems often involve sophisticated algorithms and communication protocols to manage the interplay between vastly different computational elements.
Architectural Challenges in Hybrid Systems
Designing and implementing hybrid computing architectures present significant challenges. The primary hurdle lies in the inherent incompatibility of different computing paradigms. Digital, quantum, and biological systems operate under vastly different physical principles and require specialized hardware and software. Efficient data transfer and communication between these disparate components are crucial, requiring the development of novel interfaces and protocols.
Furthermore, error correction and fault tolerance mechanisms must be adapted to accommodate the unique error profiles of each paradigm. For instance, quantum systems are highly susceptible to noise, requiring sophisticated error mitigation strategies that differ significantly from those used in digital systems. Finally, the development of appropriate programming models and software tools to effectively utilize the capabilities of hybrid systems is a crucial ongoing effort.
Conceptual Design of a Hybrid Computing Architecture
Imagine a layered architecture. The base layer is a massively parallel biological computing system, perhaps a network of engineered cells performing computations using DNA or other biomolecules. This layer excels at handling complex, high-dimensional data. The second layer is a neuromorphic chip acting as an intermediary. It receives processed data from the biological layer and performs pattern recognition and preliminary analysis.
This layer’s strength lies in its ability to extract meaningful features from raw data, significantly reducing the computational load on the upper layers. The top layer consists of a conventional digital processor that orchestrates the overall computation, receives refined data from the neuromorphic layer, and performs final calculations and decision-making. This layer is responsible for precise computations, control, and communication with the external world.
Data flows between layers via specialized interfaces designed to convert data formats and manage communication protocols. This layered approach allows each paradigm to operate optimally within its strengths, resulting in a synergistic computational system that surpasses the capabilities of individual components. The design aims for a modular architecture, enabling flexibility and scalability by adding or removing layers as needed, depending on the specific computational task.
This allows for the efficient use of computational resources and facilitates the optimization of the system for different applications.
Societal Implications and Ethical Considerations
The shift away from digital computing, while promising immense technological advancements, necessitates a careful consideration of its societal and ethical ramifications. The potential benefits are undeniable – increased energy efficiency, novel computational capabilities, and perhaps even solutions to problems currently intractable for digital computers. However, this transition also introduces significant uncertainties and challenges that demand proactive engagement from researchers, policymakers, and the public.
A responsible approach requires a thorough examination of both the potential upsides and downsides of this technological paradigm shift.The development and deployment of powerful alternative computing technologies present a complex ethical landscape. These technologies, ranging from bio-inspired computing to quantum computing, possess the potential to revolutionize various aspects of life, from medicine and materials science to artificial intelligence and national security.
However, this power also brings with it the risk of misuse, unintended consequences, and the exacerbation of existing societal inequalities. The very nature of these technologies, often opaque and difficult to understand, adds another layer of complexity to the ethical considerations.
Potential Societal Impacts of a Shift Away from Digital Computing
A transition to alternative computing paradigms could lead to significant societal changes. For example, the development of more energy-efficient computing could mitigate the environmental impact of data centers, a considerable source of carbon emissions. Conversely, the concentration of expertise and resources in new computing technologies could exacerbate existing economic disparities, creating a digital divide between those who have access to and benefit from these advancements and those who do not.
The potential for new forms of automation driven by alternative computing could also lead to significant job displacement in certain sectors, requiring proactive measures for workforce retraining and adaptation. Consider, for instance, the impact of automation in manufacturing – a shift to alternative computing could accelerate this trend, requiring societal adaptation to manage its effects.
Ethical Implications of Developing and Deploying Alternative Computing Technologies
The ethical implications are multifaceted and require careful consideration. The potential for misuse of powerful alternative computing technologies, such as in the development of autonomous weapons systems or sophisticated surveillance technologies, is a significant concern. Furthermore, questions of data privacy and security become even more critical as the underlying computational principles become more complex and less transparent. The challenge of ensuring equitable access to the benefits of these technologies, preventing their monopolization by powerful entities, is also paramount.
The potential for unintended consequences, due to the inherent complexity of these systems, necessitates rigorous testing and evaluation before widespread deployment. For example, unforeseen biases in algorithms trained on alternative computing platforms could perpetuate and even amplify existing societal biases.
Potential Risks and Benefits of Widespread Adoption of Non-Digital Computing Methods
The widespread adoption of non-digital computing methods presents both significant risks and potential benefits. On the benefit side, we could see advancements in areas like personalized medicine, materials discovery, and environmental monitoring, all enabled by the unique computational capabilities of alternative systems. However, risks include the potential for unforeseen vulnerabilities, the difficulty of verifying the correctness of computations, and the potential for malicious actors to exploit these new technologies.
Consider the example of a bio-inspired computing system used in medical diagnostics – a failure in the system could have severe consequences. Therefore, robust security measures and rigorous testing protocols are essential to mitigate these risks.
Potential Ethical Guidelines for the Responsible Development and Use of Alternative Computing
It is crucial to establish clear ethical guidelines to govern the development and use of alternative computing technologies. These guidelines should prioritize:
- Transparency and explainability: Algorithms and systems should be designed to be as transparent and understandable as possible, allowing for scrutiny and accountability.
- Equitable access: The benefits of alternative computing should be accessible to all members of society, regardless of socioeconomic status or geographic location.
- Privacy and security: Robust measures should be implemented to protect user data and prevent unauthorized access or misuse.
- Environmental sustainability: The environmental impact of alternative computing technologies should be carefully considered and minimized.
- Safety and reliability: Thorough testing and evaluation should be conducted to ensure the safety and reliability of these technologies before widespread deployment.
- Accountability and oversight: Mechanisms for accountability and oversight should be established to address potential harms and misuse.
The quest to transcend the limitations of digital computing is leading us down incredible paths. From harnessing the power of quantum mechanics to mimicking the intricacies of biological systems, the future of computation is brimming with possibilities. While challenges remain – scalability, energy efficiency, ethical considerations – the potential rewards are immense. This exploration into alternative computing paradigms is not just about technological advancement; it’s about reshaping our understanding of computation itself and its potential to solve some of humanity’s greatest challenges.
The journey has just begun, and it promises to be an exciting one!