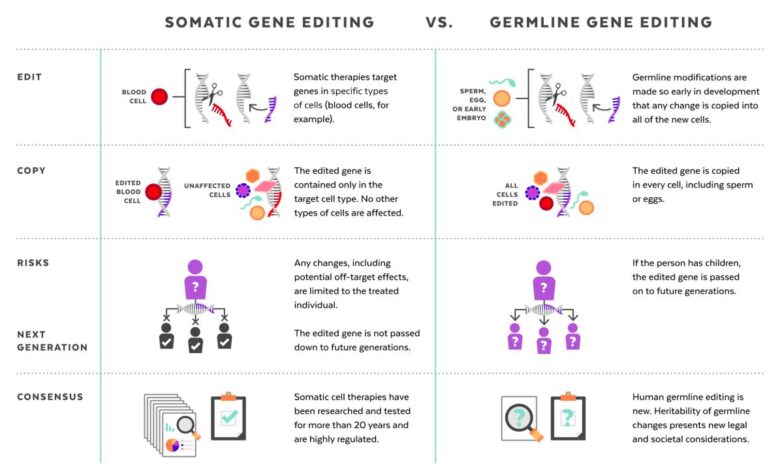
Gene Editing Drugs From Lab to Clinic at Lightning Speed
Gene editing drugs are moving from lab to clinic at lightning speed, revolutionizing medicine as we know it. This incredible pace is fueled by breakthroughs in technologies like CRISPR-Cas9, TALENs, and ZFNs, offering unprecedented potential to treat previously incurable genetic diseases. We’re seeing clinical trials yielding promising results for a range of inherited and acquired conditions, raising hopes for a future where genetic diseases are a thing of the past.
But alongside this exciting progress come crucial ethical considerations and challenges that need careful consideration.
The rapid advancement is driven by a confluence of factors: improved gene editing tools, a deeper understanding of human genetics, and streamlined regulatory pathways. While CRISPR-Cas9 currently dominates the field due to its relative simplicity and efficiency, other technologies are also contributing to this medical revolution. However, the journey from bench to bedside isn’t without its hurdles. Concerns around off-target effects, long-term consequences, and equitable access to these potentially life-saving treatments remain significant challenges that need to be addressed proactively.
The Rapid Advancement of Gene Editing Therapies
The field of gene editing has exploded in recent years, transitioning from a promising research area to a rapidly developing therapeutic landscape. This remarkable progress is fueled by technological breakthroughs, leading to a surge in clinical trials and the potential for revolutionary treatments for a wide range of previously incurable diseases. The speed of this translation from bench to bedside is unprecedented in the history of medicine.
Key Technological Breakthroughs
Several key technological advancements have propelled the rapid translation of gene editing from research labs into clinical trials. Improvements in delivery systems, particularly viral vectors like adeno-associated viruses (AAVs), have significantly enhanced the efficiency and safety of delivering gene editing tools to target cells. Furthermore, the development of more precise and efficient gene editing technologies themselves, alongside sophisticated screening and selection methods for identifying and isolating successfully edited cells, have been crucial.
The development of robust analytical tools allowing for precise assessment of editing efficiency and off-target effects has also been essential in accelerating the clinical translation process, ensuring safety and efficacy.
Timeline of Gene Editing Drug Development and Approval
While a complete timeline requires constant updating due to the rapid pace of advancements, some key milestones highlight the accelerating progress. The first clinical trial using zinc finger nucleases (ZFNs) for gene editing began in 2010. CRISPR-Cas9 technology quickly emerged as a dominant player, entering clinical trials in the mid-2010s. The first approval of a gene editing therapy (for sickle cell disease and beta-thalassemia) marked a pivotal moment, underscoring the clinical viability of these approaches.
Numerous other gene editing therapies are currently in various stages of clinical trials, focusing on diverse conditions like cancer, inherited retinal diseases, and HIV. This rapid pace of clinical trials reflects the significant investment and collaborative efforts across academia, industry, and regulatory bodies.
Comparison of Gene Editing Technologies
The following table compares three major gene editing technologies used in clinical settings:
Technology | Mechanism | Advantages | Limitations |
---|---|---|---|
Zinc Finger Nucleases (ZFNs) | Uses engineered zinc finger proteins to bind specific DNA sequences, guiding a nuclease to create a double-stranded break. | Relatively high specificity compared to earlier methods. | Design and production can be complex and expensive; potential for off-target effects. |
Transcription Activator-Like Effector Nucleases (TALENs) | Similar to ZFNs, but uses TAL effector proteins for DNA binding, offering greater flexibility in target site selection. | Easier design and construction than ZFNs; improved targeting compared to ZFNs. | Still relatively complex and costly to produce; potential for off-target effects, though reduced compared to ZFNs. |
CRISPR-Cas9 | Uses a guide RNA molecule to direct the Cas9 nuclease to a specific DNA sequence, creating a double-stranded break. | Simple and inexpensive to design and produce; high efficiency; easily adaptable to target various genes. | Potential for off-target effects; delivery challenges remain; ethical concerns surrounding germline editing. |
Clinical Applications of Gene Editing Drugs
The rapid translation of gene editing technologies from the laboratory to clinical trials marks a pivotal moment in medicine. These powerful tools offer unprecedented opportunities to treat a wide range of diseases previously considered incurable, paving the way for personalized therapies tailored to individual genetic profiles. This section will delve into the specific applications of gene editing drugs, highlighting both successes and challenges.
Gene editing therapies are being actively explored for a diverse array of both inherited and acquired conditions. Inherited diseases, caused by mutations present from birth, are particularly well-suited to gene editing approaches that aim to correct the underlying genetic defect. Acquired diseases, on the other hand, develop later in life and may benefit from gene editing strategies that target specific cells or pathways involved in disease progression.
Inherited Diseases Targeted by Gene Editing
Inherited blood disorders, such as sickle cell disease and beta-thalassemia, are at the forefront of gene editing clinical trials. These conditions result from mutations in the gene responsible for producing hemoglobin, the protein that carries oxygen in red blood cells. Gene editing strategies aim to correct these mutations, restoring normal hemoglobin production and alleviating the debilitating symptoms of these diseases.
For example, CRISPR-Cas9-based therapies have shown promising results in clinical trials, demonstrating significant improvements in patients’ health. The technology allows for precise targeting and correction of the faulty gene within hematopoietic stem cells (the cells that give rise to blood cells), resulting in the production of healthy red blood cells.
Acquired Diseases Targeted by Gene Editing, Gene editing drugs are moving from lab to clinic at lightning speed
Beyond inherited disorders, gene editing holds promise for treating acquired diseases like cancer. Certain cancers are driven by specific genetic alterations that promote uncontrolled cell growth. Gene editing approaches can be used to target and disrupt these oncogenes, effectively halting tumor growth. Additionally, gene editing is being explored for the treatment of viral infections, such as HIV, by modifying immune cells to make them resistant to viral infection.
This approach aims to create a functional cure by eliminating the virus from the body or significantly reducing its viral load.
The rapid advancement of gene editing drugs from lab to clinic is both exciting and terrifying. We need strong, ethical regulations to guide this explosive field, and that got me thinking about effective governance. Learning from past mistakes is crucial, which is why I recommend checking out this insightful article on lessons from Justin Trudeau’s failings in Canada – the need for responsible leadership applies equally to cutting-edge science like gene editing.
Ultimately, the speed of these advancements demands equally swift and considered policy responses.
Successful Clinical Trials and Efficacy
Several clinical trials have demonstrated the efficacy and safety of gene editing drugs. For instance, in trials for sickle cell disease, patients treated with CRISPR-Cas9-based gene editing experienced a significant reduction in painful vaso-occlusive crises and an increase in the levels of fetal hemoglobin, a type of hemoglobin that is not affected by the sickle cell mutation. Similarly, clinical trials for beta-thalassemia have shown impressive results, with patients achieving transfusion independence after gene editing therapy.
These successes highlight the transformative potential of gene editing in treating previously intractable diseases.
Ethical Considerations and Potential Risks
The rapid advancement of gene editing therapies necessitates careful consideration of ethical implications and potential risks.
The following points summarize key ethical concerns and potential risks:
- Off-target effects: Gene editing tools, while highly specific, may unintentionally modify other genes, leading to unforeseen consequences. Rigorous safety testing is crucial to minimize these off-target effects.
- Long-term consequences: The long-term effects of gene editing therapies are not fully understood. Comprehensive follow-up studies are necessary to assess the safety and durability of these treatments over time.
- Accessibility and equity: The high cost of gene editing therapies raises concerns about accessibility and equity. Ensuring that these treatments are available to all patients who could benefit, regardless of their socioeconomic status, is a critical ethical consideration.
- Germline editing: The ethical implications of germline editing, which involves modifying genes in reproductive cells, are particularly complex. Changes made to germline cells are heritable and can affect future generations, raising concerns about unintended consequences and the potential for misuse.
Regulatory Landscape and Market Potential: Gene Editing Drugs Are Moving From Lab To Clinic At Lightning Speed
The breathtaking pace of gene editing drug development necessitates a thorough understanding of the regulatory hurdles and market dynamics shaping this revolutionary field. Navigating the complex approval processes in major global markets and identifying key players are crucial for predicting the future growth and market share of these transformative therapies. This section delves into these critical aspects, offering insights into the regulatory landscape and projecting the market potential of gene editing drugs.The regulatory pathways for gene editing therapies are stringent and vary significantly across different jurisdictions.
This reflects the inherent complexity and potential risks associated with altering the human genome. The approval process typically involves multiple phases of clinical trials, rigorous data analysis, and comprehensive safety assessments.
The rapid advancement of gene editing drugs is truly amazing; it feels like we’re on the verge of a medical revolution. It makes you wonder about the speed of societal change, too – are we moving as fast on critical issues like political reform? I mean, check out this article about how never trump republicans are still shouting is anyone listening , and it’s a stark contrast to the breakneck pace of gene editing breakthroughs.
It’s fascinating how different sectors progress at such wildly varying speeds; hopefully, the urgency felt in medical science can inspire similar progress in other vital areas.
Regulatory Pathways in Major Global Markets
The United States, Europe, and China represent the largest and most influential markets for gene editing therapies. In the US, the Food and Drug Administration (FDA) oversees the approval process, employing a risk-based approach that considers the specific gene editing technology, the target disease, and the potential adverse effects. The European Medicines Agency (EMA) follows a similar rigorous pathway in Europe, emphasizing patient safety and efficacy.
China’s National Medical Products Administration (NMPA) also has a robust approval process, although it may differ in certain aspects from the US and European regulatory frameworks. Each agency has its own specific requirements for clinical trial design, data submission, and post-market surveillance. For instance, the FDA’s emphasis on demonstrating a clear benefit-risk profile is mirrored by the EMA’s focus on robust clinical evidence and long-term safety data.
The NMPA’s process, while becoming increasingly stringent, may exhibit differences in timelines and specific regulatory requirements compared to Western counterparts.
Key Players in Gene Editing Therapy Development and Commercialization
Several pharmaceutical giants and innovative biotech companies are at the forefront of gene editing therapy development. Crispr Therapeutics, Intellia Therapeutics, and Editas Medicine are prominent examples of companies focusing on CRISPR-Cas9 technology. Large pharmaceutical companies such as Novartis and Pfizer are also actively involved, leveraging their extensive resources and expertise in drug development and commercialization. Major research institutions, including universities and government-funded research centers, play a vital role in the fundamental research and preclinical development of gene editing technologies.
These collaborations between industry and academia are crucial for accelerating the translation of scientific discoveries into clinical applications. For example, the partnership between the Broad Institute and several pharmaceutical companies has yielded significant advancements in CRISPR-based therapies.
It’s amazing how fast gene editing drugs are progressing; they’re practically teleporting from the lab to clinical trials! Meanwhile, the political world is a bit more chaotic – I just saw this crazy news story about a dem senator hit with a bar complaint for openly threatening the Supreme Court , which is a stark contrast to the precision of gene editing.
Seriously, the speed of these medical advancements is incredible compared to the snail’s pace of some political processes.
Market Potential and Projected Growth
The market for gene editing drugs is expected to experience exponential growth in the coming decade. The current market size is relatively small, but the potential is enormous, driven by the growing prevalence of genetic disorders and the increasing demand for effective treatments. Several factors contribute to this projected growth, including technological advancements leading to improved efficacy and safety, increasing investment in research and development, and the expanding regulatory approvals for gene editing therapies.
Projected Market Growth (Bar Chart Description)
A bar chart illustrating the projected market growth over the next 10 years would show a steep upward trend. The chart would have years (2024-2034) on the horizontal axis and market size (in billions of US dollars) on the vertical axis. The bars would progressively increase in height, reflecting the anticipated exponential growth. For instance, a conservative estimate might show a market size of approximately $5 billion in 2024, rising to $50 billion or more by 2034.
This projection reflects the anticipated approvals of multiple gene editing therapies for various diseases, increasing market penetration, and the overall expansion of the market. This projection is supported by market research reports from reputable firms that analyze trends in the pharmaceutical and biotechnology sectors. For example, a report by a leading market research firm might show a similar trend of exponential growth, based on their analysis of clinical trial data, regulatory approvals, and investment trends.
Challenges and Future Directions
The breathtaking speed at which gene editing therapies are transitioning from the lab to clinical trials is undeniably exciting. However, this rapid progress also highlights significant hurdles that must be addressed to ensure these powerful tools are both effective and accessible for patients worldwide. The journey from promising research to widespread clinical application is fraught with challenges that require innovative solutions and careful consideration.The successful translation of gene editing technologies into routine clinical practice hinges on overcoming several key obstacles.
These range from the purely scientific, such as achieving precise targeting and minimizing off-target effects, to the broader societal considerations of cost, equity, and ethical implications.
Cost-Effectiveness and Accessibility of Gene Editing Therapies
The high cost of developing and manufacturing gene editing therapies presents a significant barrier to widespread access. Current gene therapies often involve complex manufacturing processes and individualized treatment approaches, leading to exorbitant price tags. This makes them largely inaccessible to many patients, particularly in low- and middle-income countries. Strategies to reduce production costs, such as developing more efficient manufacturing processes and exploring alternative delivery methods, are crucial.
Furthermore, innovative payment models and collaborative efforts between pharmaceutical companies, governments, and insurance providers are needed to ensure equitable access. For example, exploring tiered pricing models based on a patient’s ability to pay, or government subsidies for specific gene therapies targeting life-threatening conditions, could help bridge the accessibility gap.
Target Specificity and Minimizing Off-Target Effects
A critical challenge lies in achieving precise gene editing. While CRISPR-Cas9 and other gene editing technologies offer remarkable precision, off-target effects—unintended edits at other genomic locations—remain a concern. These off-target edits can have unpredictable consequences, potentially leading to adverse events. Ongoing research focuses on improving the specificity of gene editing tools, including the development of enhanced guide RNAs and improved delivery systems.
Rigorous preclinical testing and careful monitoring of patients in clinical trials are essential to identify and mitigate off-target effects. The development of sophisticated screening methods to detect off-target edits is also vital. For instance, whole-genome sequencing is increasingly used to assess the extent of off-target edits in clinical trials.
Expanding the Therapeutic Scope of Gene Editing
The potential applications of gene editing extend far beyond the currently approved therapies. While significant progress has been made in treating inherited monogenic disorders, the field is poised to revolutionize the treatment of more complex diseases. Cancer therapy is a prime example, with gene editing offering the potential to engineer immune cells to target cancer cells more effectively (CAR T-cell therapy) or to directly edit cancer cells to eliminate their malignant properties.
Gene editing also holds immense promise for treating infectious diseases, such as HIV, by targeting viral genes or enhancing the immune response. Furthermore, gene editing technologies are being explored for neurodegenerative disorders, such as Alzheimer’s and Parkinson’s diseases, aiming to correct genetic defects or modulate gene expression to prevent or slow disease progression. For instance, research is underway to use gene editing to correct mutations in genes linked to Alzheimer’s disease in animal models, showing promising results in reducing amyloid plaque formation.
Roadmap for Future Research and Development in Gene Editing
The future of gene editing requires a multi-pronged approach encompassing fundamental research, technological advancements, and ethical considerations. A potential roadmap for future research and development could include:
- Improving Target Specificity and Reducing Off-Target Effects: Develop novel gene editing tools with enhanced specificity and fidelity, explore improved guide RNA design, and refine delivery systems to minimize off-target edits.
- Developing Novel Delivery Systems: Investigate safer and more efficient delivery methods, including viral vectors with improved tropism and non-viral delivery systems such as nanoparticles or lipid-based carriers, to ensure targeted delivery of gene editing tools to specific cells and tissues.
- Expanding Therapeutic Applications: Explore the potential of gene editing for treating a broader range of diseases, including complex disorders such as cancer, infectious diseases, and neurodegenerative disorders. This includes developing personalized therapies tailored to individual patient genetic profiles.
- Addressing Cost and Accessibility Issues: Develop cost-effective manufacturing processes, explore innovative payment models, and establish equitable access strategies to ensure that gene editing therapies are available to all patients who could benefit.
- Establishing Robust Regulatory Frameworks: Develop clear and comprehensive regulatory guidelines for the development and clinical application of gene editing therapies to ensure patient safety and ethical considerations are addressed.
- Addressing Ethical and Societal Concerns: Conduct thorough ethical analyses of gene editing technologies, including germline editing, and establish clear ethical guidelines for research and clinical applications.
Patient Access and Societal Impact
The breathtaking speed at which gene editing therapies are transitioning from the lab to the clinic presents incredible opportunities, but also significant challenges. Ensuring equitable access and mitigating potential societal risks are paramount to realizing the full potential of this revolutionary technology while avoiding exacerbating existing health disparities. The cost of these therapies, coupled with complex regulatory hurdles and potential unforeseen long-term effects, necessitates careful consideration of their impact on healthcare systems and society as a whole.The implications of gene editing therapies on healthcare systems are multifaceted.
The high cost of development and manufacturing, combined with the individualized nature of many treatments, will likely create significant financial strain. This could lead to unequal access, where only the wealthy can afford these life-altering treatments, thus widening the existing healthcare gap. Furthermore, the integration of these therapies into existing healthcare infrastructure requires substantial investment in training, infrastructure, and monitoring systems.
The sheer complexity of gene editing procedures also demands highly specialized medical personnel, potentially creating shortages in already strained healthcare systems.
Healthcare System Strain and Access Disparities
The exorbitant cost of gene editing therapies poses a significant barrier to access. For example, the cost of CAR T-cell therapy, a type of gene editing treatment for certain cancers, can easily exceed $500, This price tag effectively excludes many patients, particularly those lacking adequate insurance coverage or residing in countries with less robust healthcare systems. This creates a critical ethical dilemma: should access be based solely on ability to pay, or should society find ways to ensure equitable distribution of these potentially life-saving treatments?
This necessitates innovative financing models, such as government subsidies, tiered pricing structures based on income, or risk-sharing agreements between manufacturers and payers. The potential for “gene editing tourism,” where individuals travel to countries with more accessible or affordable treatments, also needs consideration.
Ethical Considerations and Social Equity
Gene editing raises profound ethical questions. Concerns about “designer babies,” the potential for unintended off-target effects, and the long-term consequences of altering the human germline demand careful consideration and robust regulatory frameworks. Social equity is another critical concern. The potential for gene editing to exacerbate existing social inequalities, by making certain enhancements or treatments available only to the wealthy, must be proactively addressed.
The use of gene editing technologies for non-therapeutic purposes, such as enhancing physical or cognitive abilities, also raises ethical dilemmas regarding fairness and potential social stratification.
Strategies for Equitable Access and Risk Mitigation
To ensure equitable access and mitigate potential societal risks, a multi-pronged approach is needed. This includes establishing transparent and equitable pricing mechanisms, developing robust regulatory frameworks that prioritize patient safety and ethical considerations, and investing in research and development to reduce the cost of gene editing therapies. Public education initiatives are also crucial to foster informed public discourse and address misconceptions surrounding gene editing technologies.
International collaborations are necessary to ensure global access and avoid a scenario where only wealthier nations benefit from these advancements.
Key Recommendations: Establish a global collaborative effort to develop ethical guidelines and regulatory frameworks for gene editing; Implement innovative financing models to ensure equitable access based on need, not ability to pay; Invest heavily in research to reduce the cost and increase the accessibility of gene editing therapies; Launch public education campaigns to promote informed discussion and address public concerns.
The breathtaking speed at which gene editing therapies are transitioning from the laboratory to clinical application marks a pivotal moment in medical history. While challenges remain in terms of cost, accessibility, and potential risks, the potential benefits for patients suffering from debilitating genetic disorders are immense. Continued research, ethical dialogue, and responsible regulation are vital to ensure that these powerful technologies are developed and deployed in a way that benefits all of humanity.
The future of gene editing is bright, promising a future where we can rewrite the code of life to cure disease and improve human health.